For several years now, Advanced Space has analyzed orbital dynamics for the Lunar Gateway. Supporting the Gateway’s Mission Design and Navigation (MDNAV) team, located primarily at NASA’s Johnson Space Center, these analyses have helped inform station requirements; develop concepts of operations; plan for contingencies; and assist with other planning activities. Our experience with operating the CAPSTONE™ mission in the unique near-rectilinear halo orbit (NRHO) environment has enabled us to help NASA understand the best ways to operate spacecraft in that orbit. These results have been shared with the Gateway team and at industry conferences.
How the Orbit Works and Why We Are Using It
NRHO is an interesting orbit. If you could observe a vehicle in NRHO from a distance while the Moon orbits the Earth and Earth remains stationary, the spacecraft’s path would appear to make a crown-shaped path around the planet as it made multiple loops around the Moon (image below, left). If you were on Earth and could watch the vehicle’s path while the Moon remained directly overhead, the spacecraft would appear to circle the Moon in a long ellipse (halo), with one end of its orbit much closer to the Moon than the other (image below, right).
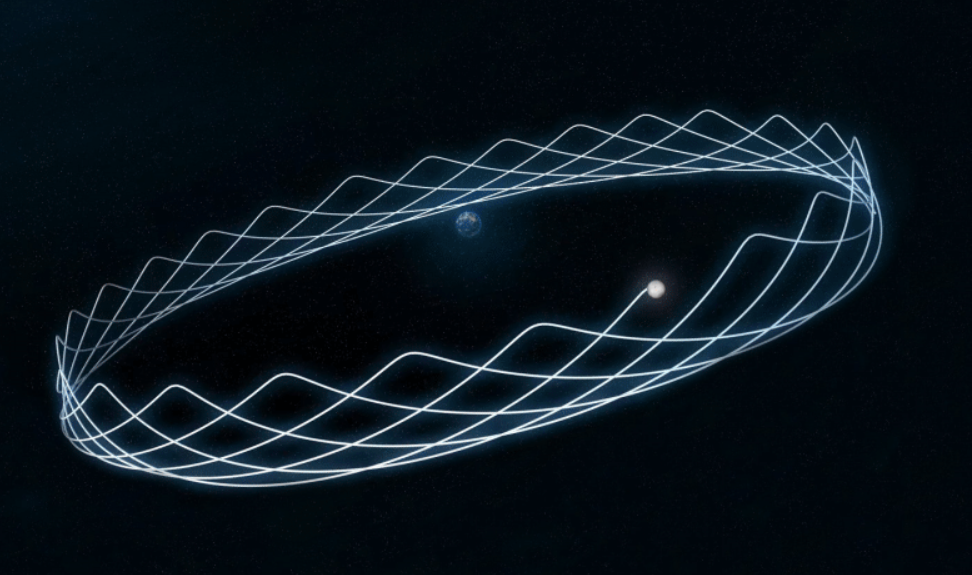
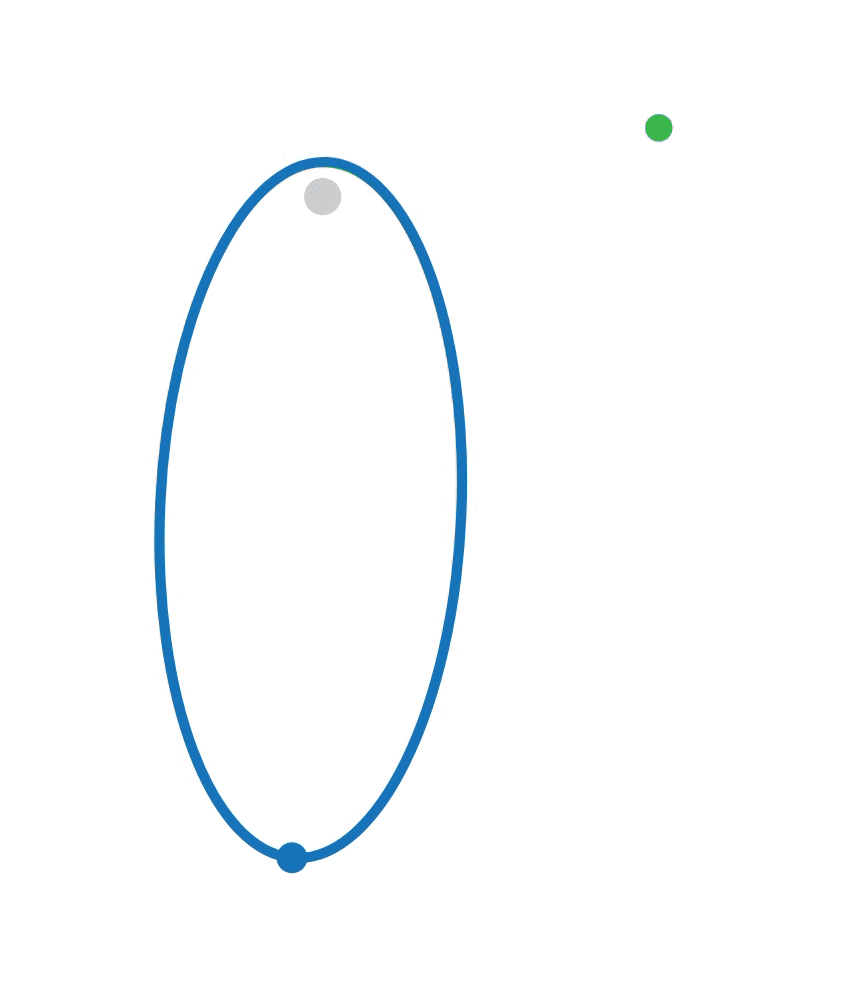
Vehicles in the NRHO are acted upon by gravity from the Moon, Earth, and the Sun. Because of the orbit’s “halo” shape, a spacecraft can nearly always be in communication with Earth while using it (unlike the Apollo Program’s low lunar orbits, which experienced communication blackouts when the spacecraft went behind the Moon). By carefully timing its orbit insertion and station-keeping propulsion burns, a spacecraft or station in NRHO also can avoid eclipses by the Earth, which enables solar panels to continue functioning. Both the lack of communication blackout and the constantly operating solar power make the orbit useful to the Lunar Gateway. CAPSTONE therefore serves as a pathfinder for the Gateway.
The multiple sources of gravity influencing NRHO also make it slightly unstable, making it easier for a vehicle to enter the orbit inbound from Earth, drop out of it to descend to the Moon, or exit the orbit to head back home. This instability also requires regular, small correction burns to keep a vehicle in the orbit and to ensure that it continues avoiding eclipses. All of these factors influence how much propellant a spacecraft or space station needs to maintain its position in NRHO, which is of great interest to NASA, as propellant mass¹ affects mission and spacecraft designs.
1) In orbital mechanics, propellant is most frequently called “delta-V” for the change (delta or ∆) in velocity it provides.
Lessons Learned
Through the analyses we’ve conducted, Advanced Space has learned the following:
Making Corrections for the Long Cruise
Near Rectilinear Halo Orbits, the target of the Lunar Gateway, are slightly unstable and therefore require minimal delta-V for insertion. This instability means that insertion using highly efficient, low-thrust systems is a viable and attractive option; however, entering orbit also may require the spacecraft to approach the maneuver on a dynamically sensitive trajectory using multiple passes close to the Moon. Our analyses investigated a low-thrust NRHO insertion from a lunar transfer that spirals out from Earth for this type of approach. We identified correction maneuver and navigation strategies that are robust to initial state, navigation, and maneuver execution errors prior to and through insertion. In addition, we demonstrated that these strategies allow the spacecraft to transition to nominal NRHO station keeping after insertion, without the need for specially designed clean-up maneuvers or a significant amount of additional delta-V.
(Source: “Navigation and Mission Design for Low-Thrust Insertion into Near Rectilinear Halo Orbits” by Ethan W. Kayser, et al.)
Improving Station-keeping Practices to Reduce Propellant Costs
Most station-keeping strategies for spacecraft in NRHOs extrapolate the current best-estimate of a trajectory forward and design a maneuver to reach a target that exists multiple revolutions into the future. Our studies quantified the range of outcomes (dispersions) on an extrapolated trajectory and examined the effect of targeting strategy changes on these dispersions. A better understanding of the true future state dispersions for NRHO station-keeping could be used to develop station-keeping strategies that lower delta-V costs over current baseline strategies.
(Source: “An Analysis of Downstream Uncertainty in NRHO Stationkeeping Strategies” by Michael R. Thompson, et al.)
Taking the Scenic Route to the Moon
Ballistic lunar transfers (BLTs) can be used to transfer from Earth to NRHOs in an efficient manner. BLTs are a type of low-energy transfer in which a spacecraft launches 1-2 million kilometers away from the Earth (where the Sun’s gravity perturbation becomes dominant), then returns to Earth with a larger radius of perigee than before and a different geocentric orbit plane. When designed with the proper geometry, it is possible to choose the perigee to coincide with the Moon’s orbit, bringing the spacecraft near the Moon. For many three-body target orbits, it is possible to design the transfer so that it arrives at the target orbit with very little insertion delta-V required. In the ideal case, the transfer is ballistic (zero deterministic delta-V) after launch. This type of transfer is being considered for delivering the Logistics Module, lander elements, and other cargo to the Lunar Gateway.
(Source: “Survey of Ballistic Lunar Transfers to Near Rectilinear Halo Orbit,” by Nathan L. Parrish, et al.)
Example BLTs to NRHO without a targeted lunar flyby. Each family of orbits is evaluated over one month to show the evolution of the family over time.
Pluses and Minuses of NRHOs
The characteristics of NRHOs that make them desirable for cislunar operations also introduce navigation challenges. The orbit’s large apolune distance (farthest point in the orbit from the Moon) provides excellent coverage of the lunar south pole, and its Earth-facing plane ensures constant line-of-sight with ground stations. However, the nearly perpendicular motion to the Earth-spacecraft line results in poor observability for determining range biases (potential errors in measuring distance), and the necessarily low perilune altitude (the point in the orbit closest to the Moon) results in highly dynamic and sensitive perilune passages. Preliminary work indicates that range biases and state estimates near apolune and perilune are unintuitively related to range and range-rate measurement quality.
(Source: “Range Biases, Measurement Noise, and Perilune Accuracy in Near Rectilinear Halo Orbit Navigation,” by Connor Ott, et al.)
We Have Results…Now What?
We have studied multiple ballistic lunar transfer (BLT) trajectories² from Earth launch to NRHO insertion. BLTs have favorable properties for uncrewed launches to orbits in the vicinity of the Moon, such as dramatically reducing requirements for spacecraft delta-V. Our results derive from a detailed set of related mission design and navigation studies: maneuver placement, tracking cadence, and statistical delta-V requirements for various navigation state errors. Monte Carlo analyses that simulate BLT navigation with various DSN tracking schedules have been presented. Those various analyses address a range of questions related to navigating a spacecraft on a BLT to an NRHO and inform future missions.
(Source: “Ballistic Lunar Transfers to Near Rectilinear Halo Orbit: Operational Considerations,” by Nathan L. Parrish, et al.)
2) A ballistic trajectory is one where no substantial additional force is applied to the vehicle flying it after its initiation, like a cannonball being fired, rising, and falling to Earth.
Tracking Measurements, Cleaning Up Insertion Maneuvers, Phasing Trajectory Tracking
Advanced Space conducted a high-fidelity simulation of spacecraft orbit determination in an NRHO, as well as investigations of various aspects of NRHO operations, including orbit insertion and long-horizon orbit maintenance. When propagated in a high-fidelity physics model, an NRHO is quasi-periodic in the Earth-Moon rotating frame, and the orbital plane is approximately perpendicular to the line-of-sight from Earth. This geometry leads to navigational challenges because Earth-based range and range-rate measurements cannot measure the main orbital motion directly. Radio-based (range/rate) measurements perform best when the spacecraft has some motion in the line-of-sight direction. As such, some aspects of the system can be difficult to observe at times. Additionally, NRHOs are characterized by large variations in the distance to the Moon: the radius of the NRHO ranges from approximately 3,500 km at perilune to about 71,000 km at apolune. These significant differences cause the acceleration experienced by the spacecraft to vary by 2-3 orders of magnitude within a single revolution. Further navigational complications arise from the limited control authority available to a spacecraft with solar electric propulsion (SEP), such as the Power and Propulsion Element (PPE) of the Lunar Gateway. It is apparent that the SEP control acceleration is considerably less than the gravity of the Moon and Earth. If the spacecraft’s mathematical orbit determination filter fails to track the spacecraft, there is a potential risk that the spacecraft’s path could diverge beyond the point of recoverability by the SEP thrusters. That is, there is some amount of spacecraft state error beyond which the spacecraft’s limited control authority is inadequate to return to the nominal NRHO without large corrections or the use of an alternative propulsion system such as RCS thrusters.
In this study, we found that a two-maneuver strategy can successfully clean up errors associated with NRHO insertion, which often occurs in an area that is highly sensitive dynamically. The cost of insertion cleanup varies with the location of the maneuvers, with the total cleanup delta-V ranging from 0.056 meters per second (m/s) to over 16 m/s. It was shown that the area-to-mass ratio of a spacecraft in NRHO has a large impact on the annual station-keeping cost due to solar radiation pressure (SRP) perturbations. We also found that the phasing of navigation tracking passes within the NRHO can be more important than the cumulative tracking time, partially due to the significant nonlinearities associated with region around perilune.
(Source: “Near Rectilinear Halo Orbit Determination with Simulated DSN Observations,” by Nathan L. Parrish, et al.)
Plans for the Future
There are many areas of interest that require more investigation in the future. The Lunar Gateway is expected to host crewed spacecraft frequently such as Orion or Starship, resupply modules, service modules, and other visiting vehicles. Understanding short-term rendezvous and docking, loitering, and relative motion near NRHO will be key to performing those activities successfully.
Lessons learned from CAPSTONE™ navigation data to inform the Lunar Gateway have been very beneficial. The next step is to introduce a navigation framework that considers the various perturbations from Gateway – such as orbital maintenance maneuvers (OMMs), desaturation maneuvers, docking/undocking, vents, active reaction control system (RCS) maneuvering burns – and to model those perturbations accurately to determine how well they can be observed.
The nominal transfers to the Moon’s vicinity for the Artemis program have been well understood. Now the focus is directed toward providing support for operations, specifically looking at contingency (i.e., emergency) scenarios. Various abort trajectories need to be generated at several locations along the nominal transfer route to go back to Earth safely at any points during the mission. We still have a lot of work to do!